B2.1 Membranes and membrane transport
B2.1.1 The Phospholipid Bilayer
The keywords:
- Ampiphatic: having a hydrophilic (water-loving) and a hydrophobic end (water-hating)
- Phospholipid: the backbone molecule of the cell membrane. Made from a phosphate group and two fatty acid chains, joined through glycerol.
The basis of all membranes, either cell membranes or the membranes that surround organelles; is that they are formed from a phospholipid bilayer (two layers of the molecule we call phospholipids).
Phospholipids are ampiphatic, meaning they have a hydrophilic head (phosphate) and two hydrophobic tails (fatty acids).
In the bilayer, phospholipids are arranged so that the hydrophobic tails point towards each other, and the hydrophilic heads point outwards.
Discuss: Why are the phospholipids arranged in this way?
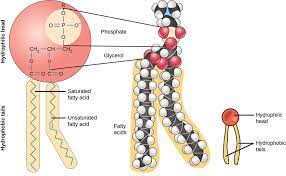
Discuss the image above showing the chemical structure. Which parts of the molecule have charged or polar groups? Which parts of the molecular are hydrophobic / hydrophilic?
B2.1 Lipid bilayers as Barriers
The hydrophilic tails limit the permeability of hydrophilic molecules (such as charged ions like sodium). Larger molecules also have difficulty passing as the phospholipids are closely packed.
This is why protein channels are necessary to allow ions and larger molecules like glucose to enter the cell membrane.
Free energy graphs
Free energy is a measure of how much energy a molecule has. Generally, molecules try to reach a low level of free energy.
As a molecule crosses the phospholipid bilayer, if it is hydrophobic it will interact with the hydrophobic tails and reduce its level of free energy. If the same molecule were hydrophilic, it would increase its level of free energy as it would be repelled by the hydrophobic tails.
Discuss the graph below: Identify the most hydrophilic, and the most hydrophobic drug.
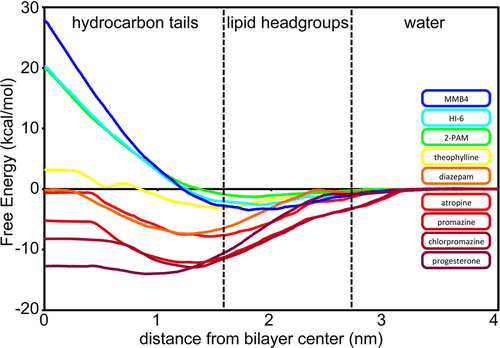
B2.1.3 Simple diffusion across a membrane
Diffusion is the spreading out of particles from a high concentration to a low concentration, not requiring energy.
Simple diffusion across the phospholipid bilayer is possible for small non-polar molecules, such as gases.
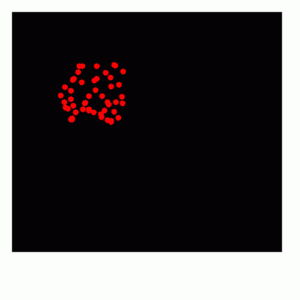
List: Gases which are likely to cross a cell membrane in this way.
B2.1.4 Integral and Peripheral Proteins
The position of proteins in the cell membrane reflects their function and hydrophilic properties.
Peripheral proteins are embedded in the outside of the bilayer, and are hydrophilic. They are often involved in cell recognition (combined with carbohydrate groups to form molecules called glycoproteins.
Integral proteins pass through the membrane from one side to the other, and have hydrophobic groups on the parts inside the membrane, and hydrophilic groups on the parts of their molecules that protude outside of the membrane. They are involved in the transport of molecules across membranes (as channel proteins or protein pumps).
B2.1.5 Movement of water molecules across membranes by osmosis and the role of aquaporins.
Osmosis is the movement of water from low to high solute concentration, across a membrane.
Although water is a partially permeable molecule, it is small enough to pass through bilayer.
Some cells have additional protein channels called aquaporins to enhance the membrane permeability. This is particularly useful in the cells that line the nephrons in the kidney, which need to reabsorb water from filtered blood.
Discuss: Where else in the human body would you expect cell membranes to contain aquaporins?
B2.1.6 Channel protein for facilitated diffusion
Integral proteins can be used for the diffusion of polar or medium sized molecules, from a high concentration to a low concentration. These are called channel proteins.
Channel proteins can be gated, which means this diffusion can be controled by a physical barrier which may be open or closed.
Channel proteins can be specific to an ion because of their diameter and properties. Sodium channels found in the nervous system, are a good example.
B2.1.7 Pump proteins for active transport
Other integral proteins use the energy from ATP (adenosine tri-phosphate) to move molecules by active transport.
Active transport is the movement of molecules across a membrane from a low to a high concentration, agains the concentration gradient, requiring energy.
In order to achieve this movement, pump proteins change their conformation or shape. This requires energy, and moves the required nutrient to the other side of the membrane.
Protein pumps are designed to move specific molecules the same way. For example, the sodium-potassium pumps in a nerve cell alway pump sodium into a membrane, and potassium out.
B2.1.8 Selectivity in membrane permeability
A membrane will vary in terms of its selective permeability by the number and nature of protein channels and pumps. For example, if a membrane in the respiratory system contains many cloride channels, it will be more permeable to chloride. If it contains no channel proteins and no protein pumps that transport magnesium, it will have a low permeability to magnesium.
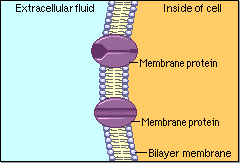
Overall the transport across membranes can be summarised:
- Simple non polar or small molecules – go straight through by diffusion
- Water – travels by osmosis
- Charged or medium molecules – require either a channel protein (down a concentration gradient), or a protein pump (up concentration gradient)
Summary diagram (note carrier protein is the same thing as a protein pump).

B2.1.9 Structure and fuction of glycoproteins and glycolipids
Imagine two hunan cheek cells side by side on the endothelial layers of the mouth. Do they ´recognise´ each other as belonging to the same body?
Yes they do!
Carbohydrate groups combine either with protins (called glycoproteins), or with phospholipids (glycolipids), to form complex molecules which can interact with the molecules of any other cell which comes into contact with the membrane, to identify self (part of the same body), or alien (such as bacteria).
Q) Apart from cell recognition of cells from the same tissue, how else could cell recognition be useful?
B2.1.10 Fluid mosaic model of membrane structure
Combining all the elements discussed, a speculative model has been designed to represent the probable arrangement of all cell membranes.
Task: Working with a partner, identify the components labeled A-I.

(answers found below).
B2.1.11 Relationship between fatty acid composition of bilayers and their fluidity
The fatty acid chains of the phospholipid molecules that form the bilayer may be saturated (no double bonds), or unsaturated (contain double bonds).
Fluidity refers to how easy it is for molecules to move around int
Saturated fatty acid chains are straighter and longer, and so can pack together more tightly and reduce membraine fluidity. They also make the cell membrane thicker.
Unsaturated fatty acid chains are bent and shorter, and so pack together more loosely, which increases membrane fluidity.
Discuss: What effect would you expect freezing cold water temperatures to have on the membrane fluidity of fish living in the Arctic waters? How would you expect the ratio of saturated to unsaturated fatty acids to compare with those of tropical fish?
Answers:
- A: Phospholipid A1 phosphate, A2 fatty acid chains
- B: Glycolipids
- C: Glycoproteins
- D: Carbohydrate group
- E: Cholesterol
- F: Peripheral protein
- G: Integral protein
B2.1.12 Cholesterol and membrane fluidity
Cholesterol also helps to regulate memrane fluidity. Cholesterol is also ampiphatic, and found within the phospholipid bilayer.
B2.1.13 Membrane fluidity and the formation of vesicles
Membranes with high levels of fluidity can even break off parts of themselves, to form vesicles (little bags made from membrane). This can happen inwards or outwards, and is an important part of transporting large molecules.
This is how white blood cells ingest bacteria, a process called endocytosis. This is also how many unicellular organisms feed.
This is also how your stomach secretes digestive enzymes, in litle vesicles in a process called exocytosis.
B2.1.14 Gated ion channels in neurons.
Passive transport may occur through integral protein channels that open and close. These are gated ion channels.
When a nerve impulse passes along the longest part of a neuron (nerve cells), the axon, the impulse passes because of gated sodium channels that open in response to a change in voltage.
Potassium channels are involved in the recovery of a neuron after an impulse has passed.
Q) How are sodium and potassium ions specific to individual channels?
The diameter of gated ion channels matches the diameter of a specific ion. A ball and chain mechanism, where subunits of the channel protein block the passage of ions until the voltage changes, is used in the design of sodium and potassium ion channels.

B2.1.15 Sodium-Potassium pumps as an example of exchange transporters
When energy is used to transport ions across an integral protein, this called active transport and the protein is called a pump.
The most common pump in cell membranes is the sodium-potassium pump, which exchanges sodium for potassium. This means that sodium is pumped across the membrane going in one direction only, and potassium is pumped across the membrane in the opposite direction
Specifically, three sodium ions are pumped in one direction, and two potassium ions are pumped in the opposite direction.
B.2.1.16 Sodium-dependent glucose cotransporters as an example of indirect active transport
The urine of a healthy humam being does not contain glucose. This is because the cells of the kidney (in a part called the proximal tubule) actively reabsorb glucose against the concentration gradient.
- Sodium-potassium pump uses ATP to establish a gradient where there is a high concentration of sodium outside the cell.
- Co-transporter proteins in the membrane combine sodium with glucose, to move both passively across the membrane. The sodium moves back down the concentration gradient, bringing the glucose with it. Because the ATP is not used directly to pump glucose, this is called secondary or indirect active transport.
B2.1.17 Adhesion of cells to form tissues
Ever wondered how neighbouring cells in the same tissues stick together? Well, partly this is due to cell surface proteins that are embedded in the cell membranes of neighbouring cells, that bond together.
These proteins are called Cell Adhesion Molecules (CAM)
The extra-cellular matrix is a system of protein filaments that can attach to cells at specific anchor points. This also helps to hold cells together.
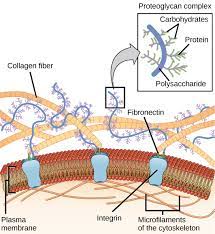